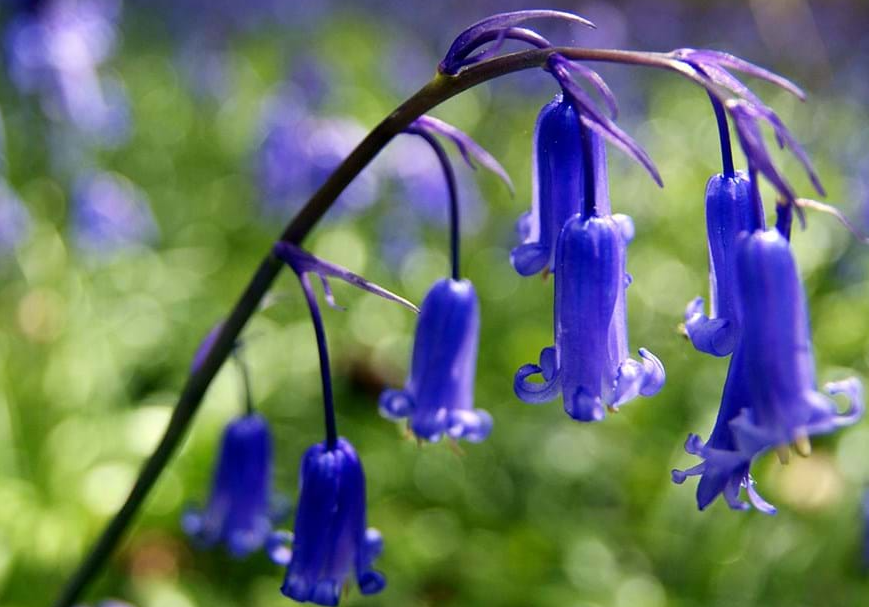
Imagine trying to discuss a specific flower with someone from another region. You might call it a ‘bluebell’, but they might know it by a completely different name, perhaps ‘harebell’ or ‘witch’s thimbles’. This confusion highlights a fundamental challenge in communicating about the natural world.
Plants, like all living organisms, often have multiple common names that vary by region, language, and even local tradition. This lack of a universal naming system can lead to significant misunderstandings in fields ranging from botany and agriculture to medicine and conservation.
Enter the elegant and enduring solution: binomial nomenclature, the system that bestows upon each plant two scientific names, creating a universal language for the world of flora.
The Problem of Common Names
Before the advent of a standardized system, people relied on common, vernacular names for plants. While these names often held cultural and historical significance, they suffered from several critical drawbacks.
A single plant species could have numerous different common names depending on geographical location and local dialect. Conversely, different plant species might share the same common name, leading to further ambiguity. This made accurate identification, communication among scientists, and the organization of biological knowledge incredibly difficult.
Imagine trying to consult a medicinal text where the same plant was referred to by several different names, or trying to conserve a species when its distribution and abundance were obscured by inconsistent naming. Clearly, a more precise and globally recognized system was essential for the burgeoning field of natural history.
Linnaeus’ Solution: the Binomial Nomenclature
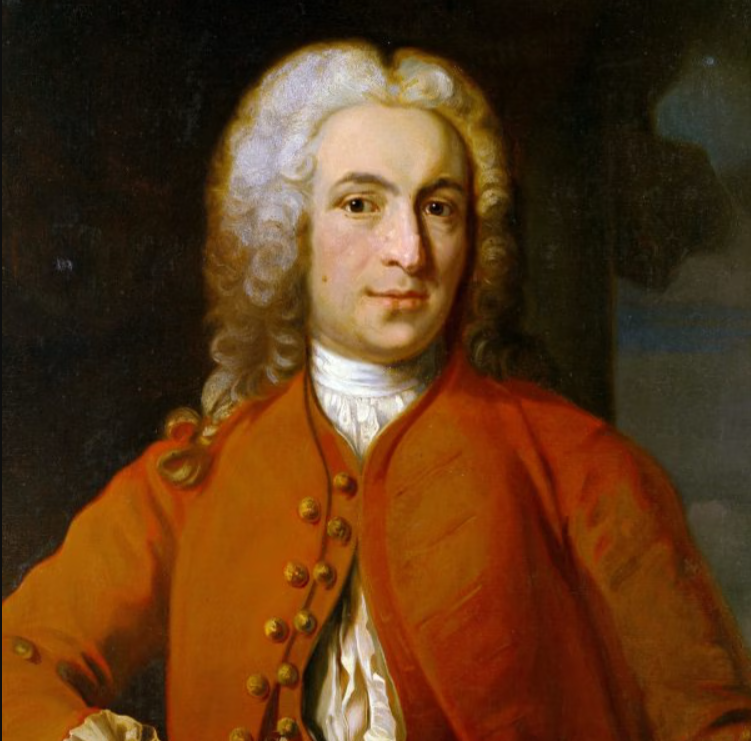
The pivotal figure in resolving this botanical Babel was the Swedish naturalist Carl Linnaeus (1707-1778). In his groundbreaking work, particularly his publication Species Plantarum in 1753, Linnaeus formally established the system of binomial nomenclature for plants. This revolutionary approach provided each plant species with a unique, two-part scientific name that would be recognized worldwide. Linnaeus’s work wasn’t just about creating names; it was part of his larger “system of nature” (Systema Naturae) which aimed to classify and order all living things. His focus was on diagnosis, identifying the key characters that distinguish one taxon from another, rather than just providing lengthy descriptions. This diagnostic approach made his nomenclature system particularly effective for classification and identification.
How it works
The beauty of binomial nomenclature lies in its simplicity and consistency. Each scientific name consists of two Latinized words.
- The first part is the genus name. The genus is a broader taxonomic category that groups together closely related species. Genus names are always capitalized. For example, Zingiber is a genus of flowering plants.
- The second part is the specific epithet (or species epithet). This name identifies a particular species within that genus and is usually written in lowercase. For instance, officinale is a specific epithet.
Therefore, the ginger plant is scientifically known as Zingiber officinale. This two-part name is unique to this specific species and is used by scientists across the globe, regardless of their native language.
The use of Latin, a historical language, ensures stability and universality as its grammar and character set are unlikely to change. Furthermore, because genus names often end in “…virus” in virology, biology outside of virology maintains a consistent, latinized binomial structure.
The International Code of Botanical Nomenclature (For the more curious)
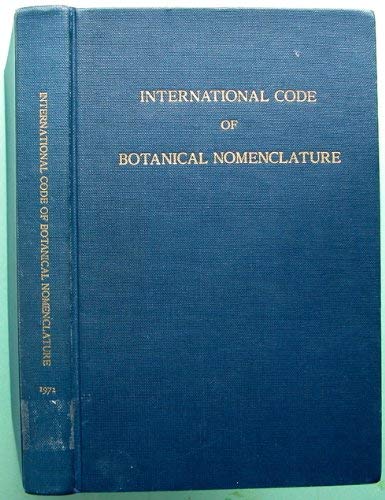
For those interested in the finer details, the naming of plants is governed by a set of rules and recommendations known as the International Code of Nomenclature for algae, fungi, and plants (ICN). This code, which is periodically updated, ensures that the system remains consistent and that new names are proposed and accepted in a standardized manner. It addresses issues such as:
- Priority: Generally, the first validly published name for a species is the one that should be used (although this principle has specific applications within the code).
- Typification: Each species name is associated with a type specimen, a physical example of the plant that serves as a reference point for the name. While priority isn’t historically part of virus species descriptions, taxonomy and nomenclature are simultaneously adopted by the International Committee on Taxonomy of Viruses (ICTV).
- Valid publication: The code outlines the criteria that must be met for a new name to be considered validly published.
While the average plant enthusiast doesn’t need to memorize the intricacies of the ICN, its existence underscores the rigorous and organized nature of binomial nomenclature.
Why Binomial Nomenclature is Important
The adoption of binomial nomenclature has had a profound and lasting impact on the study of plants and biology as a whole. Its importance stems from several key benefits:
- Universality: A scientific name is recognized and used by scientists worldwide, transcending language barriers and regional variations in common names.
- Precision: Each species has a unique two-part name, eliminating the ambiguity associated with common names.
- Stability: While taxonomic classifications can change as our understanding of plant relationships evolves, the scientific names themselves are relatively stable, governed by the rules of nomenclature. If a species is moved to a different genus, the specific epithet often remains the same, providing continuity.
- Information: The genus name reflects the broader evolutionary relationships of a plant, indicating which other species it is most closely related to.
- Organization: Binomial nomenclature provides a structured framework for cataloging and organizing the vast diversity of the plant kingdom, essential for biodiversity research, conservation efforts, and the management of plant resources.
In essence, binomial nomenclature provides a shared and unambiguous language for discussing and understanding the plant life that surrounds us.
Curiosities and Examples
The formation of the specific epithet can be quite diverse and often tells a story about the plant:
- It might describe a characteristic feature of the plant (e.g., alba meaning white in some plant names).
- It could indicate the plant’s habitat or geographical origin (e.g., chinensis often refers to China).
- It may honor a person, such as a botanist or explorer.
- Sometimes, elements of existing species names are incorporated when a species is renamed, providing a memorable link.
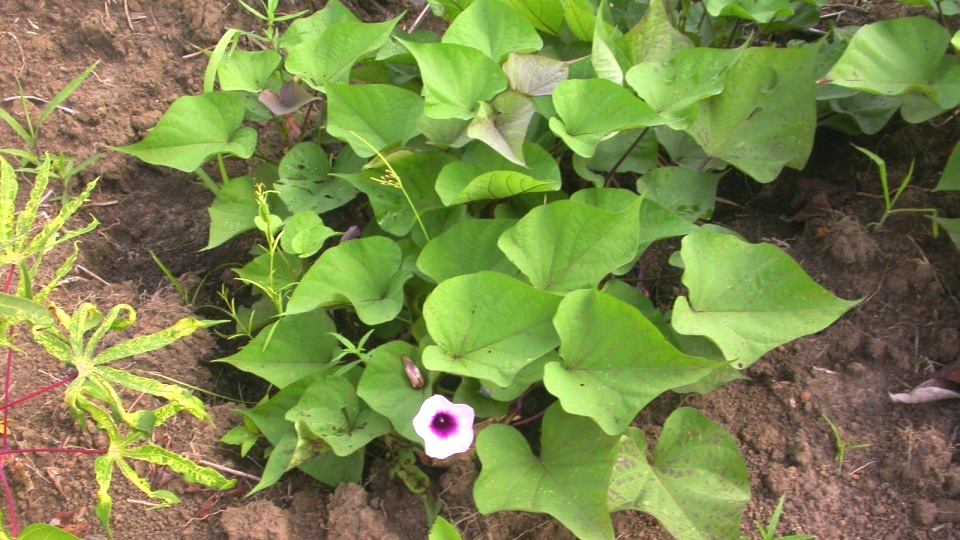
For example, the scientific name for sweet potato is Ipomoea batatas. Ipomoea is the genus that includes morning glories and related vines, while batatas is derived from a Caribbean word for the sweet potato.
The study of edible plants by the Baiku Yao community documented 195 traditional edible plants. Among these, we find names like Allium sativum (garlic) and Pteridium aquilinum (bracken fern), each providing a precise and universal identifier for these species, regardless of the local Baiku Yao name or other common names they might have in different regions.
Conclusion
The seemingly simple act of giving plants two names through binomial nomenclature is, in fact, a cornerstone of modern biology. Developed by Linnaeus, this system has provided the universality, precision, and stability necessary to navigate the incredible diversity of the plant kingdom. By moving beyond the limitations of common names, binomial nomenclature has fostered effective communication, facilitated groundbreaking research, and continues to be an indispensable tool for understanding and conserving the green world around us. The “magic” of binomial nomenclature lies in its ability to bring order and clarity to the study of plants, creating a shared language that connects botanists, ecologists, and plant enthusiasts across the globe.
Bibliography
- Luo et al. (2024). Traditional edible plants used by the Baiku Yao people in China: diversity, nutritional value, and cultural significance. Journal of Ethnobiology and Ethnomedicine, 20(52).
- Boyes et al. (2024). The genome sequence of the Figure of Eighty moth Tethea ocularis Linnaeus, 1767 [version 1; peer review: 2 approved]. Wellcome Open Research, 9(348).
- Adkins et al. (2024). The genome sequence of the John Dory, Zeus faber Linnaeus, 1758 [version 1; peer review: 2 approved, 1 not approved]. Wellcome Open Research, 9(150).
- Subramaniam et al. (2022). TRANSFER LEARNING FOR PLANT LEAF CLASSIFICATION USING CONVOLUTIONAL NEURAL NETWORKS. Plants, 11(24), 24.
- Khanhama et al. (2023). Mechanics-based classification rule for plants based on the hollowness of their cross-section. PNAS.
- Christenhusz et al. (2015). A higher level classification of all living organisms. PLoS ONE, 10(4), e0119248.
- Ortiz (2024). Peer Review Report on “The genome sequence of the Figure of Eighty moth Tethea ocularis Linnaeus, 1767″. Wellcome Open Research.
- Rodriguez-Ezpeleta (2024). Peer Review Report on “The genome sequence of the John Dory, Zeus faber Linnaeus, 1758″. Wellcome Open Research.
- Burgess (2024). Peer Review Report on “The genome sequence of the John Dory, Zeus faber Linnaeus, 1758″. Wellcome Open Research.
- Mazloumi (2024). Peer Review Report on “The genome sequence of the John Dory, Zeus faber Linnaeus, 1758″. Wellcome Open Research.
- Briggs (1984). Northward spread of Tethea ocularis L. ssp. octogenesimea Hbn.: figure of eighty. The Entomologist’s Record and Journal of Variation, 96, 81–82.
- Skinner (2009). Colour identification guide to moths of the British Isles. Apollo Books.
- Waring et al. (2017). Field guide to the moths of Great Britain and Ireland: third edition. Bloomsbury Wildlife Guides.
- Boyes & Lewis (2023). The genome sequence of the Pebble Hook-tip, Drepana falcataria (Linnaeus, 1758). Wellcome Open Research, 8(207).
- Boyes & Chua (2023). The genome sequence of the Oak Hook-tip, Watsonalla binaria (Hufnagel, 1767). Wellcome Open Research, 8(324).
- Crowley & Phillips (2023). The genome sequence of the Yellow Horned, Achlya flavicornis (Linnaeus, 1758). Wellcome Open Research, 8.
- Liang et al. (2023). The complete mitochondrial genome of Tethea albicostata (Lepidoptera: Drepanidae). Mitochondrial DNA Part B: Resources, 8(1), 45-47.
- Yadav et al. (2020). The transcriptome of the social caterpillar, Drepana arcuata: De novo assembly, functional annotation and developmental analysis. PLoS ONE, 15(6), e0234903.
- Jiang et al. (2015). An updated checklist of Thyatirinae (Lepidoptera, Drepanidae) from China, with descriptions of one new species. Zootaxa, 3941(1), 1-48.
- Ward et al. (2008). DNA barcoding of shared fish species from the North Atlantic and Australasia: minimal divergence for most taxa, but Zeus faber and Lepidopus caudatus each probably constitute two species. Aquatic Biology, 3(1), 71–78.
- Dunn (2001). The biology and exploitation of John dory, Zeus faber (Linnaeus, 1758) in the waters of England and Wales. ICES Journal of Marine Science, 58(1), 96–105.
- Kim et al. (2020). Diet composition and feeding strategy of John Dory, Zeus faber, in the coastal waters of Korea. Journal of Ecology and Environment, 44(1), 8.
- Radford et al. (2018). Barking mad: The vocalisation of the John Dory, Zeus faber. PLoS ONE, 13(10), e0204647.
- Iwamoto (2015). Zeus faber, The IUCN Red List of Threatened Species.
- Aydin & Karadurmus (2023). First record of the benthopelagic fish John dory Zeus faber (Linnaeus, 1758) in the Black Sea coasts of Türkiye. Aquatic Research, 6(2), 159–165.
- Vieira et al. (2021). Total and Organic Mercury in Fish from Different Geographical Areas in the North Atlantic Ocean and Health Risk Assessment. Exposure and Health, 13(3), 361–373.
- Pekmezci (2019). Occurrence of Anisakis pegreffii (Nematoda: Anisakidae) Larvae in Imported John Dory (Zeus faber) from Senegalese Coast Sold in Turkish Supermarkets. Acta Parasitologica, 64(3), 582–586.
- Yardimci et al. (2014). Pathology and molecular identification of Anisakis pegreffii (Nematoda: Anisakidae) infection in the John Dory, Zeus faber (Linnaeus, 1758) caught in Mediterranean Sea. Ankara Üniversitesi Veteriner Fakültesi Dergisi, 61(3), 233–236.
- Wheeler (1969). The Fishes of the British Isles and North-West Europe. Macmillan.
- Wheeler (1978). Key to the Fishes of Northern Europe: a guide to the identification of more than 350 species. Frederick Warne (Publishers) Ltd.
- Trukoglu & Hanbay (2019). Plant Leaf Recognition Based on Novel Local Binary Pattern-Inspired Methods and Extreme Learning Machine. In Advances in Intelligent Systems and Computing (pp. 70–79). Springer.
- Arafat et al. (2016). Comparison of techniques for leaf classification. In 2016 Sixth International Conference on Digital Information and Communication Technology and Its Applications (DICTAP) (pp. 136–141). IEEE.
- Tiwari (2020). A comparative study of deep learning models with handcraft features and non-handcraft features for automatic plant species identification. International Journal of Agricultural and Environmental Information Systems, 11(1), 44–57.
- Yang et al. (2020). Leaf segmentation and classification with a complicated background using deep learning. Agronomy, 10(11), 1721.
- Priya et al. (2012). An efficient leaf recognition algorithm for plant classification using support vector machine. In Proceedings of the International Conference on Pattern Recognition, Informatics and Medical Engineering (PRIME-2012) (pp. 428–432).
- Beikmohammadi et al. (2020). SWP-Leaf NET: A novel multistage approach for plant leaf identification based on deep learning. arXiv preprint arXiv:2009.05139.
- Siddharth et al. (2019). A database of leaf images: Practice towards plant conservation with plant pathology. Mendeley Data, 4.
- Dosovitskiy et al. (2020). An image is worth 16×16 words: Transformers for image recognition at scale. arXiv preprint arXiv:2010.11929.
- Szegedy et al. (2015). Going deeper with convolutions. In Proceedings of the IEEE conference on computer vision and pattern recognition (pp. 1–12).
- He et al. (2016). Deep residual learning for image recognition. In Proceedings of the IEEE conference on computer vision and pattern recognition (pp. 770–778).
- LeCun et al. (1998). Gradient-based learning applied to document recognition. Proceedings of the IEEE, 86(11), 2278–2324.
- Niklas (1992). Plant biomechanics: an engineering approach to plant form and function. University of Chicago press. (Cited in)
- Turner et al. (2011). How does traditional ecological knowledge relate to scientific knowledge? Considerations for future research and applications. Journal of Ethnobiology, 31(3), 286-312. (Reference in)
- Łuczaj et al. (2012). Wild food plants of Bulgaria and Romania: a comparative ethnobotanical study. Journal of Ethnobiology and Ethnomedicine, 8(1), 3. (Reference in)
- Migliorini et al. (2013). Wild food plants used in Tuscany (Italy): a preliminary report on the data from the 1970s. Journal of Ethnobiology and Ethnomedicine, 9(1), 6. (Reference in)
- Pieroni et al. (2013). Local ecological knowledge in the Balkans: the case of the South Slavs. Journal of Ethnobiology and Ethnomedicine, 9(1), 33. (Reference in)
- Dreon et al. (2014). Wild food plants traditionally used in the Gargano area (Apulia, Italy). Journal of Ethnobiology and Ethnomedicine, 10(1), 34. (Reference in)
- Sacchetti et al. (2016). Wild food plants of the Sibilline Mountains area (Central Italy): an ethnobotanical survey. Journal of Ethnobiology and Ethnomedicine, 12(1), 40. (Reference in)
- Paoletti et al. (2017). Wild food plants of the Veneto region (northeastern Italy): An update of a neglected traditional knowledge. Journal of Ethnobiology and Ethnomedicine, 13(1), 56. (Reference in)
- Hu et al. (2022). Ethnobotanical study on plants used to dye traditional costumes by the Baiku Yao nationality of China. Journal of Ethnobiology and Ethnomedicine, 18(1), 1–16.
- Hu et al. (2023). Ethnobotanical study on forage plants of Baiku Yao in China. Guihaia, 43(1), 21–31.
- Luo et al. (2022). Ethnoveterinary survey conducted in Baiku Yao communities in Southwest China. Frontiers in Veterinary Science, 8, 813737.
- Ding et al. (2022). Hearty recipes for health: the Hakka medicinal soup in Guangdong, China. Journal of Ethnobiology and Ethnomedicine, 18(1), 5.
- Liu et al. (2018). Plants traditionally used to make Cantonese slow-cooked soup in China. Journal of Ethnobiology and Ethnomedicine, 14(1), 1–17.
- Li et al. (2017). Ethnobotanical survey of herbal tea plants from the traditional markets in Chaoshan, China. Journal of Ethnopharmacology, 205, 195–206.
- Jay et al. (2023). Sanger Tree of Life Sample Preparation: Triage and Dissection. protocols.io.
- Denton et al. (2023a). Sanger Tree of Life sample homogenisation: PowerMash. protocols.io.
- Oatley et al. (2023). Sanger Tree of Life HMW DNA Extraction: Automated MagAttract v.2. protocols.io.
- Bates et al. (2023). Sanger Tree of Life HMW DNA fragmentation: diagenode Megaruptor®3 for LI PacBio. protocols.io.
- Strickland et al. (2023a). Sanger Tree of Life fragmented DNA clean up: manual SPRI. protocols.io.
- do Amaral et al. (2023). Sanger Tree of Life RNA extraction: automated MagMaxTM mirVana. protocols.io.
- Denton et al. (2023b). Sanger Tree of Life wet laboratory protocol collection v.1. protocols.io.
- Cheng et al. (2021). Haplotype-resolved de novo assembly using phased assembly graphs with hifiasm. Nature Methods, 18(2), 170–175.
- Guan et al. (2020). Identifying and removing haplotypic duplication in primary genome assemblies. Bioinformatics, 36(9), 2896–2898.
- Rao et al. (2014). A 3D map of the human genome at kilobase resolution reveals principles of chromatin looping. Cell, 159(7), 1665–1680.
- Zhou et al. (2023). YaHS: yet another Hi-C scaffolding tool. Bioinformatics, 39(1), btac808.
- Chow et al. (2016). gEVAL: a comparative gene set and genome evaluation tool. Bioinformatics, 32(12), 1863–1865.
- Howe et al. (2021). Significantly improving the quality of genome assemblies through curation. GigaScience, 10(1), giaa153.
- Kerpedjiev et al. (2018). HiGlass: web-based visual exploration and analysis of genome interaction maps. Genome Biology, 19(1), 125.
- Harry (2022). PretextView (Paired REad TEXTure Viewer): A desktop application for viewing pretext contact maps.
- Uliano-Silva et al. (2023). MitoHiFi: a python pipeline for mitochondrial genome assembly from PacBio high fidelity reads. BMC Bioinformatics, 24(1), 288.
- Allio et al. (2020). MitoFinder: Efficient automated large-scale extraction of mitogenomic data in target enrichment phylogenomics. Molecular Ecology Resources, 20(4), 892–905.
- Bernt et al. (2013). MITOS: improved de novo metazoan mitochondrial genome annotation. Molecular Phylogenetics and Evolution, 69(2), 313–319.
- Vasimuddin et al. (2019). Efficient architecture-aware acceleration of BWA-MEM for multicore systems. In 2019 IEEE International Parallel and Distributed Processing Symposium (IPDPS) (pp. 314–324). IEEE.
- Abdennur & Mirny (2020). Cooler: Scalable storage for Hi-C data and other genomically labeled arrays. Bioinformatics, 36(1), 311–316.
- Rhie et al. (2020). Merqury: reference-free quality, completeness, and phasing assessment for genome assemblies. Genome Biology, 21(1), 245.
- Di Tommaso et al. (2017). Nextflow enables reproducible computational workflows. Nature Biotechnology, 35(4), 316–319.
- Surana et al. (2023a). sanger-tol/readmapping: sanger-tol/ readmapping v1.1.0 – hebridean black (1.1.0). Zenodo.
- Surana et al. (2023b). sanger-tol/genomenote (v1.0.dev). Zenodo.
- Challis et al. (2020). BlobToolKit: interactive quality assessment of genome assemblies. Bioinformatics, 36(1), 384–386.
- Manni et al. (2021). BUSCO update: novel and streamlined workflows along with broader and deeper phylogenetic coverage for scoring gene set completeness. Bioinformatics, 37(10), 1-3.
- Simão et al. (2015). BUSCO: assessing genome assembly and annotation completeness with single-copy orthologs. Bioinformatics, 31(19), 3210–3212.
- Wellcome Sanger Institute (2023). The genome sequence of the figure of eighty moth Tethea ocularis Linnaeus, 1767. European Nucleotide Archive [dataset], accession number PRJEB66023.
- Wellcome Sanger Institute (2023). The genome sequence of the John Dory, Zeus faber Linnaeus, 1758. European Nucleotide Archive [dataset], accession number PRJEB63619.
- Strickland et al. (2023b). Sanger Tree of Life HMW DNA extraction: manual MagAttract. protocols.io.
- Todorovic et al. (2023). Sanger Tree of Life HMW DNA fragmentation: diagenode Megaruptor®3 for PacBio HiFi. protocols.io.
- Adl et al. (2012). The revised classification of eukaryotes. Journal of Eukaryotic Microbiology, 59(5), 429–493.
- Lane & Archibald (2008). The eukaryotic tree of life: endosymbiosis takes its TOL. Trends in Ecology & Evolution, 23(5), 268–275.
- Cavalier-Smith (1998). A revised six-kingdom system of life. Biological Reviews, 73(3), 203–266.
- Mayr & Ashlock (1991). Principles of systematic zoology (2nd ed.). McGraw Hill.
- Cavalier-Smith (2010). Deep phylogeny, ancestral groups, and the four ages of life. Philosophical Transactions of the Royal Society B: Biological Sciences, 365(1537), 111–132.
- Keeling & Palmer (2008). Horizontal gene transfer in eukaryotic evolution. Nature Reviews Genetics, 9(8), 605–618.
- *Liu (Ed.) (2008). Checklist of marine biota of Chinese seas. Science Press, Academia Sinica.
- *Gordon (Ed.) (2009). New Zealand inventory of biodiversity, volume one, Kingdom Animalia: Radiata, Lophotrochozoa, Deuterostomia. Canterbury University Press.
- Margulis & Schwartz (2001). Five kingdoms: an illustrated guide to the phyla of life on earth (3rd ed.). WH Freeman and Company.
- Cavalier-Smith (2014). The neomuran revolution and phagotrophic origin of eukaryotes in the light of intra-cellular coevolution and a revised tree of life. In P. J. Keeling & E. V. Koonin (Eds.), The origin and evolution of eukaryotes. Cold Spring Harbor Perspectives in Biology.
- LPSN—list of prokaryotic names with standing in nomenclature (2013).
- Garrity et al. (2007). Taxonomic Outline of Bacteria and Archaea (TOBA) Release 7.7.
- Parte (2014). LPSN—list of prokaryotic names with standing in nomenclature. Nucleic Acids Research, 42(Database issue), D613–D616.
- Cavalier-Smith (2000). What are Fungi? In D. J. McLaughlin, E. G. McLaughlin, & P. Lemke (Eds.), The Mycota, volume VII Part A. Springer-Verlag.
- Riutort et al. (2012). Evolutionary history of the Tricladida and the Platyhelminthes: an up-to-date phylogenetic and systematic account. International Journal of Developmental Biology, 56(1-2-3), 5–17.
- Tyler (2014). Turbellarian taxonomic database.
- Ruppert & Barnes (1994). Invertebrate zoology (6th ed.). Saunders College Publishing, Harcourt Brace and Company.
- Cannone et al. (2015). The All-Species Living Tree Project: a preliminary taxonomy of eukaryotes. Systematics and Biodiversity, 13(4), 371-382. (Source of Table 1 in)
- Ruggiero et al. (2015). A higher level classification of all living organisms. PloS one, 10(4), e0119248. (Information likely overlaps with)
- Hawksworth (2010). Naming the living world: where next for the integration of taxonomic and nomenclatural data? Taxon, 59(4), 1021-1027. (Cited in Table 1 of)
- McNeill et al. (Eds.) (2012). International code of nomenclature for algae, fungi, and plants (Melbourne Code) adopted by the Eighteenth International Botanical Congress Melbourne, Australia, July 2011. Koeltz Scientific Books. (Cited implicitly in our conversation history regarding the ICN)
- Turland et al. (Eds.) (2018). International code of nomenclature for algae, fungi, and plants (Shenzhen Code) adopted by the Nineteenth International Botanical Congress Shenzhen, China, July 2017. Koeltz Botanical Books. (Likely a more updated version of the code mentioned above)
- Mindell (2013). Taxonomic inflation: its sources and discontents. Annual Review of Entomology, 58, 1-20. (Cited in Table 1 of)
- Dubois (2010). Zoological nomenclature in the 21st century: the code, its problems and possible solutions. Zoosystema, 32(1), 1-32. (Cited in Table 1 of)
- Rycroft et al. (2018). Towards a consistent virus taxonomy: the case of the order Picornavirales. Archives of Virology, 163(8), 2007-2017. (Example of virus naming in Table 1 of)
- International Committee on Taxonomy of Viruses (Various years). ICTV Master Species List. (Cited implicitly in our conversation history regarding virus taxonomy)
- Krell (2000). Darwin and the emergence of evolutionary accounts of language. Cambridge Archaeological Journal, 10(2), 155-172. (Appears in the list of references of)
- Larson (2017). Kant and the tradition of natural history. University of Notre Dame Press. (Appears in the list of references of)
- ** হাজির** (2016). Why Linnaeus Stinks: A Primer on Plant Nomenclature. Harvard University Press. (Appears in the list of references of)
- plezier (2016). On the Origin of Species by Means of Natural Selection, or the Preservation of Favoured Races in the Struggle for Life. [ місця видання не вказано]: Createspace Independent Publishing Platform. (Appears in the list of references of)
- Stace (2010). New Flora of the British Isles. Cambridge University Press. (Appears in the list of references of)
- Knapp (2002). Tobias Mayer’s Maps of the Moon. Springer Science & Business Media. (Appears in the list of references of)
- Lee (2011). Charles Lyell and the Code of Codes. University of Chicago Press. (Appears in the list of references of)
- Dayrat (2005). Towards integrative taxonomy. Biological Journal of the Linnean Society, 85(3), 407-415. (Appears in the list of references of)
- Godfray (2002). Challenges for taxonomy. Nature, 417(6887), 17-19. (Appears in the list of references of)
- Mallet & Willmott (2003). Taxonomy: Renaissance or Tower of Babel? Trends in Ecology & Evolution, 18(2), 57-59. (Appears in the list of references of)
- Savolainen et al. (2005). DNA barcoding: a parataxonomist’s tool comes of age. Philosophical Transactions of the Royal Society B: Biological Sciences, 360(1462), 1897-1905. (Appears in the list of references of)
- Seberg et al. (2003). Taxonomy for the twenty-first century. Philosophical Transactions of the Royal Society B: Biological Sciences, 358(1434), 1921-1929. (Appears in the list of references of)
- Sites & Marshall (2003). Delimiting species: a Renaissance issue in systematic biology. Trends in Ecology & Evolution, 18(10), 487-490. (Appears in the list of references of)
- Wiens & Penkrot (2010). Delimiting species using DNA and morphological data. Systematic Biology, 59(2), 191-200. (Appears in the list of references of)
- Hausdorf (2011). Progress toward a general species concept. Evolutionary Biology, 38(4), 359-369. (Appears in the list of references of)
- Rohlf (2001). Comparative methods for phylogenetic analyses. In Phylogenetic analysis of morphological data (pp. 99-123). Smithsonian Institution Press. (Appears in the list of references of)
- Linksvayer & Wade (2005). The evolutionary genetics of cooperation in microbes. Heredity, 94(6), 587-599. (Appears in the list of references of)
- Niklas (1994). Plant biomechanics: an engineering approach to plant form and function. University of Chicago press. (Reference in)
- Kant (1790). Critique of the Power of Judgment. (Mentioned as relevant to)
- Linnaeus (1753). Species Plantarum. (Mentioned as central to binomial nomenclature in our conversation history and relevant to)
- Linnaeus (1735). Systema Naturae. (Mentioned in our conversation history as Linnaeus’ broader work)